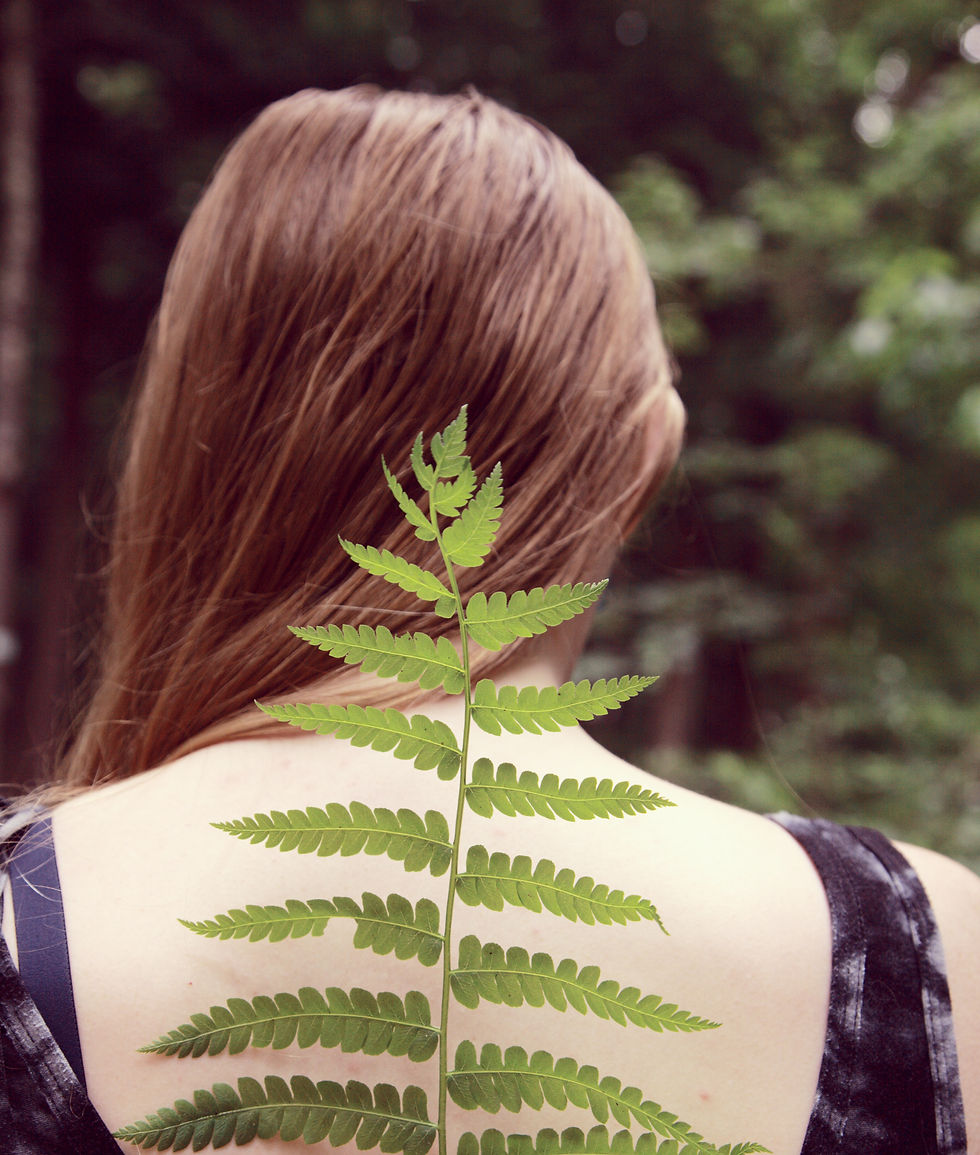
Authors: Audrey Hu and Khushee Totla
Mentor: May Sin Ke. May is currently a doctoral candidate in the Department of Oncology at the University of Oxford.
Abstract
This review paper explores recent advancements in therapies for spinal cord injury (SCI), focusing on its regenerative, neuroprotective and complementary approaches. Regenerative strategies, particularly stem-cell based therapies, offer avenues for restoring spinal cord function by differentiating into neural cells. Neural stem cells (MSCs) and induced pluripotent stem cells (iPSCs) are potential sources, and help address challenges such as tumorigenicity and complex differentiation pathways. The use of anti-inflammatory drugs is an example of neuroprotective intervention. Examples of complementary therapies include the use of artificial intelligence (AI) which helps to facilitate personalized rehabilitation plans, optimizing motor function recovery post-SCI. Despite current advancements, challenges that include patient response variability, potential side effects, and ethical considerations related to treatment protocols limit motor improvement in the long-term. Research must be continued to refine these therapies and establish their efficacy and safety in clinical practice.
Introduction
Spinal cord injury (SCI) is a current global medical issue that affects sensory, motor, and autonomic functions, often leading to permanent disabilities and a worsened quality of life (Ahuja et al., 2017). Spinal cord injuries (SCI) are any sort of injury to the spinal cord or the spinal nerves and these injuries have devastating effects upon the body (Mayo Clinic, 2023). This is because the spinal cord sends and receives messages between the brain and the body, and therefore damage to the spinal cord can cause permanent detriment to bodily functions (Mayo Clinic, 2023). Globally, SCI incidents range from 250 to 960 per million people annually, and 27 million cases are reported worldwide (Barbiellini Amidei et al., 2022). The main causes for SCI are traffic accidents, falls, and sports injuries, and because of such injuries, healthcare costs increase and long-term care requirements are needed (Lenehan et al., 2012; Liu et al., 2023). Symptoms for a SCI include partial or complete loss of sensory and motor function; bowel, bladder, and sexual dysfunction; and dysregulation of blood pressure, heart rate, and/or body temperature (World Health Organization, 2024). With over 25 million people worldwide that live with an SCI and more than 130,000 new cases of SCIs per year, it is especially critical to take measures to cure SCIs (Thuret et al., 2006).
According to the World Health Organization around 15.4 million people worldwide are living with spinal cord injury (SCI). Since 2016, the National Institutes of Health alone has spent over $530 million on SCI research, yet the average remaining years of life for patients suffering from traumatic SCI (tSCI) has not improved since the 1980s and still remains significantly below the life expectancies of people without SCI (NSCISC Facts and Figures, 2024; Dietz et al., 2022). Individuals that suffer with SCI are faced with major financial burdens, with lifetime costs that can estimate between $1 million to $4 million per person, something that varies by the severity of the injury and the necessary medical care (Chen et al., 2013). SCI also leads to substantial health challenges, including risks of secondary complications such as pressure ulcers, urinary tract infections, and respiratory issues, issues that also necessitate repeated hospitalizations and extended medical intervention (NSCISC, 2020; Chen et al., 2013). Multiple factors emphasize the urgent need for comprehensive support systems and rehabilitative strategies to enhance the quality of life for those that suffer with SCI.
SCIs can also occur in different locations of the spinal cord which will have differing effects upon the person. The cervical portion is the top portion of the body, the thoracic is the chest, the lumber is the legs, and sacral is the bowels and bladder. Also, the spinal cord has varying nerve pathways such as the corticospinal tract (voluntary movement), the spinothalamic tract (temperature information), and the dorsal column (tactile sensations) that complicate matters further (Bennett et al., 2024). Both of these make SCI biology quite complex and therefore difficult to treat as an SCI and can affect the spinal cord in different regions and intensities that alters the treatment necessary for the person.
Understanding the mechanisms behind axon growth and regeneration failure is crucial for developing new therapies and cures (Tian et al., 2023). Many therapeutic strategies have been discussed but very few have ever reached clinical trials. A total of 1149 interventional clinical trials for SCI have been done from 1996 to 2021. Of the 1,149 trials, 46.7% have been completed, 23.1% are recruiting, 10.1% are not recruiting, and 7.66% have been suspended. Of the trials that have been completed, 75.4% have no results posted while only 24.6% have results. Of the 124 completed trials with results, 95.9% met their primary endpoints but none have been applied to the general clinical population (Dietz et al., 2022). Most clinical trials that have reached stage 4 regarding SCI focus on alleviating patient discomfort and preventing further injury. A recent clinical trial involving the use of electric simulation assisted walking for the reduction of secondary injury has successfully reached stage 4 (Ontario Neurotrauma Foundation, 2013).
As for immediate treatment, it is recommended to use a brace for realignment and stabilization, and surgery to remove fractured vertebrae for better functional recovery. Then treatment currently includes physical therapy to rebuild muscle strength, occupational therapy to redevelop motor skills for daily living, vocational rehabilitation to find ways they can do paid work, educational training to find interest in areas less relying on physical ability, and recreation therapy to encourage participation in leisure activities to their level of mobility (National Institute of Neurological Disorders and Stroke, n.d.). But, current research focuses on developing therapies to improve the function of the damaged spinal cord - mainly through developing regenerative and neuroprotective therapies. A regenerative approach will focus on regenerating the broken spinal cord and replacing damaged neural cells, while a neuroprotective approach will focus on minimizing secondary injury. Although, one of the major issues stopping the development of SCI repair is the adult neuron’s inherent inability to regenerate. Young nerve cells are inherently able to reproduce, but adult nerve cells have already gone through terminal differentiation and have completely lost the ability to divide. There are two main phases in the pathophysiology of SCI: the initial mechanical injury and the following secondary damage, processes that involve complex biochemical and cellular processes such as inflammation, oxidative stress, and apoptosis (Ahuja et al., 2017). However, these methods are falling short in promoting major nerve regeneration and long-term functional improvement (Zipser et al., 2022).
Examples of current researched treatments for SCIS include neuroprotection which protects the surviving nerve cells from damage; repair and regeneration which aims at encouraging the spinal cord to repair itself through cell transplants, growth promoters, and growth scaffolds; cell therapies which replaced the damaged nerve cells with other cell types to start nerve cell growth; and neuroplasticity which retrains the nervous system to restore body function and nerve pathways through rehabilitation, electrical stimulation, and more (National Institute of Neurological Disorders and Stroke, n.d.). In fact, multiple innovative therapeutic approaches are being studied and investigated to enhance the efficacy of treatments that are stem-celled based. These have included the use of biomaterial scaffolds to support cell survival and stem cell therapy to regenerate injured cells. Additionally, other newly researched treatments include the application of exosomes to deliver bioactive molecules to the injury site (Zhang et al., 2023; Isaković et al., 2023).
Despite these new advancements, immune rejection, limited cell survival, and how complex SCI pathology is continues to prevent the clinical translation of these therapies (Pandya et al., 2014). In conclusion, SCIs are a multifaceted issue that has led to a diverse array of therapeutic strategies under investigation, and continuous research is important for overcoming current limitations and helping the outcomes for SCI patients.
This research paper will specifically focus on the neuroprotective and regenerative aspects of SCIs and complementary methods such as bio-scaffolding, cell-based therapies, exosome therapies, and more as well as the impact of these findings and their future potential as treatments for SCIs.
Results
SCI repair therapies fall under three broad categories: regenerative, neuroprotective, and complementary. The regenerative solutions included in this paper are: exosomes, growth factors, and stem cell therapies. Stem cell therapy has its own separate category because many different kinds of stem cells can be used, this review includes NSCs, MSCs, and NPCs. The neuroprotective solutions included in this paper are: anti-inflammatory drugs, TMS, and bio scaffolding. Complementary therapies include everything that does not fall under either regenerative or neuroprotective. The complementary solutions discussed in this paper are: AI generated physical rehabilitation and optogenetics.
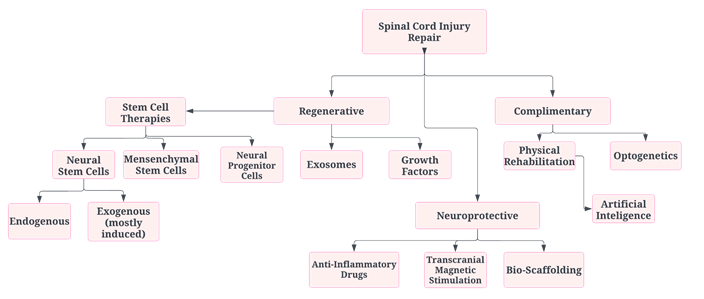
Figure 1. Overview of all SCI repair methods and therapies mentioned in this paper.
Stem Cell Therapy
Regenerative therapies are one of the main categories of therapies regarding SCI. Stem cell based therapies are considered promising cures for SCI. Stem cells have the ability to differentiate into various specialized cell types in the body (Smith & Johnson, 2023). Neural stem cells (NSCs) are multipotent stem cells that can differentiate into neuronal and neuroglial lineages (Guo et al., 2022). This makes NSCs a suitable candidate for SCI repair because the cells can differentiate into the destroyed spinal cord cells, thus regenerating the spinal cord. NSCs are overall less popular than ESCs mainly due to lack of availability (Shao et al., 2019). Though, recent efforts have improved the availability of NSCs through the exploration of alternative sources such as making induced neural stem cells (iNSCs) using human urine cells (Son et al., 2023). NSCs can only be gathered from either within the injured spinal cord (endogenous) or they can be induced/prepared outside of the injured spinal cord (exogenous) (Figure 1). Ependymal cells are the most common endogenous cells within the spinal cord. Inherently, they reside in the central canal of the spinal cord and control the production and flow of cerebrospinal fluid (CSF). Though, in the case of SCI, their ability to differentiate has been demonstrated in vitro in mice models. The experiments show that ependymal cells divide rigorously and manifest various stem cell properties (Guo et al., 2022). In a study done in 2015 by Casey et al. it was concluded that ependymal cells respond actively to SCI (Casey et al., 2021). However, more recent research reported that cells at the site of SCI are rarely ependymal cells in mice (Xue et al., 2021). These facts along with other various studies regarding ependymal cells and endogenous NSCs present a lot of conflicting evidence and results. Exogenous NSCs can be taken from either the CNS tissues or the induction of somatic cells. For a cell to be induced, it means that a somatic cell (regular body cell) was genetically reprogrammed into a state where they can undergo differentiation into other types of cells in the body. Induced NSCs and induced pluripotent stem cells (iPSCs) derived from NSCs are preferable sources for exogenous NSCs (Guo et al., 2022). This is because the accessibility of tissues within the CNS restricts their application in stem cell therapy. Current evidence has shown that iPSCs derived from NSCs have the capacity to be like endogenous NSCs and differentiate into both neuronal and neuroglial lineages.
Mesenchymal stem cells (MSCs) therapy is also an alternative option. It has received much focus in the research field due to their ability to secrete neurotrophic factors and modulate immune responses. A study was conducted where MSCs derived from human umbilical cords which were transplanted into rats with SCI (Isaković et al., 2023). The study reported a 35% improvement in motor function recovery compared to control groups. Also, human clinical trials have shown improvements in motor function and quality of life. A Phase I/II clinical trial involving 20 patients with chronic SCI, where human embryonic stem cell-derived oligodendrocyte progenitor cells were transplanted (Fan et al., 2022). The trial reported a 50% improvement in upper limb function in 45% of participants, indicating the variability of responses of human patients and the potential for positive results. However, the study also noted limitations such as variability in efficacy among patients, potential side effects related to immunosuppression, and the need for further research to optimize protocols and understand long-term outcomes.
Neural progenitor cells (NPCs) have also shown some promise, with significant functional improvements observed in animal models following the process of transplantation. A study was conducted where NPCs were transplanted into rats with SCI,resulting in a 40% improvement in locomotor function as measured by the Basco, Beattie, and Bresnahan (BBB) locomotor rating scale (Wilcox et al., 2014). The BBB locomotor rating scale measures hindlimb locomotor function in rodents to assess motor function recovery and is adapted for assessing motor function in humans as well (Scheff et al., 2002). The safety and potential efficacy in humans has been shown through early-phase clinical trials. A trial with 18 patients indicated that 60% showed notable sensory motor improvements within six months post-transplantation (Mazzini et al., 2019). However, challenges include variability in patient responses, potential risks associated with immunosuppression, and the need for further research to optimize protocols and assess long-term safety and efficacy.
Another new and promising idea for SCI treatment is exosome therapy. Exosomes, small vesicles secreted by stem cells, have been proven to enhance neural regeneration and reduce inflammation. These exosomes contain bioactive molecules such as proteins, lipids, and RNA that work to modulate cellular processes and promote tissue repair (Zhang et al., 2023). The mechanism involves exosomes delivering their cargo to recipient cells, influencing pathways related to cell survival, inflammation modulation, and tissue regeneration. This process can facilitate neural repair and protect against secondary damage following SCI (Zhang et al., 2023). Some preclinical studies involved injecting exosomes derived from MSCs into rats with SCI, leading to a 30% reduction in inflammatory markers and a 25% improvement in motor function compared to untreated groups (Schepici et al., 2023). Limitations include the need for further research to optimize dosage, timing of administration, and long-term effects in larger animal models before moving on to human trials. The goal is to harness exosomes' ability to mitigate secondary damage, promote tissue repair, and improve functionality in SCI patients (Schepici et al., 2023).
Growth Factors
Aside from stem cell therapy, the use of growth factors have been used in stimulating the regeneration of damaged neuronal cells. Growth factors have been increasingly studied in relation to SCI injury repair, however, various growth factors play different roles in repairing SCI. Neurotrophin-3, 4, and 5 (NT-3,4,5) have been recognized for their ability to protect damaged neurons and promote neuron growth/differentiation (Costăchescu et al., 2022). Nerve growth factor (NGF) can support the survival of neurons and promote the synapse (connection) formation of neurons, thus promoting neural network remodeling and motor function recovery (Yang et al., 2020). In a study done on rats, brain-derived neurotrophic factor (BNDF) was injected into rats with SCI to increase NGF levels. 7 days after this operation the rats showed a 43% increase in their BBB locomotor scale ratings (Fang et al., 2017). In a recent study NGF-NSCs were transplanted into rats with SCI to evaluate its effects on hindlimb motor function (Aderinto et al., 2023). After cell transplantation, NGF-NSC groups demonstrated significantly higher BBB locomotor scale ratings post-injury; where, the higher the BBB score is the more motor function there is. Wang et al. (2021) compare rats treated with NGF-NSCs to a placebo group also known as a sham group. 35 days post treatment, both groups had some form of improvement in their BBB ratings but the NGF-NA-SC group had a 35% higher rating than the sham group. Although the use of growth factors is very promising, unstable molecular properties and high costs severely limit their application, which remain as challenges to be addressed.
Biomaterial Scaffold
Biomaterial scaffolds, including hydrogels and nanofibers, are used to support stem cell survival as well as integration, providing an environment for cell growth and delivering therapeutic molecules to the site of injury. These scaffolds mimic the actual biological conditions in the body, making it conducive for repair and growth by creating a supportive microenvironment. This is often achieved through protein coatings that facilitate cell adhesion and maintaining the appropriate pH environment to promote cell proliferation and differentiation (Wang et al., 2018). Some research has demonstrated that biomaterial scaffolds significantly enhance the efficacy of stem cell therapy and promote tissue regeneration (Wang et al., 2018). This study was tested through having rats be treated with hydrogel scaffolds, and MSCs showed a 45% improvement in motor function compared to 20% in the MSC-only group. The findings indicate that while biomaterial scaffolds significantly enhance the efficacy of stem cell therapies for CNS injuries by improving tissue regeneration and functional recovery, further research is needed to address limitations such as the complexity of scaffold design, controlled release of bioactive molecules, biocompatibility, stability, and functional integration (Wang et al., 2018).
Neuroprotective Therapies
Neuroprotective therapies are another form of treatment for SCIs that aims to preserve and fix neuronal damage that has occurred to the spinal cord from an SCI. Strategies of neuroprotection that will be covered in the next section of this paper include surgery, the maintenance of arterial pressure, anti-inflammatory drugs, transcranial magnetic stimulation, biomaterial scaffolds, artificial intelligence, and optogenetics.
Anti-Inflammatory Drugs
An anti-inflammatory drug called N-acetylcysteine amide (NACA) was tested through a rat model of SCI (Pandya et al., 2014). This treatment resulted in a 40% reduction in inflammatory cytokines and a 50% increase in neuronal survival. Apoptosis refers to programmed cell death essential for maintaining tissue balance, while oxidative stress results from an imbalance between reactive oxygen species (ROS) and antioxidant defenses, contributing to cellular damage (Pandya et al., 2014). Targeting apoptosis and oxidative stress is critical in spinal cord injury to mitigate secondary damage and preserve neural function (Pandya et al., 2014). Yet, NACA is still in the animal model stage of testing and has not reached the clinical stage.
Transcranial Magnetic Stimulation
Transcranial magnetic stimulation (TMS) has shown some promise in modulating cortical excitability and promoting neural plasticity. Clinical studies have reported that following TMS treatment, there have been good improvements in motor function and spasticity reduction (Tazoe & Perez, 2015). A study involving 15 patients with incomplete SCI, TMS alongside rehabilitation have led to a 20% improvement in motor scores instead of rehabilitation alone. Transcranial magnetic stimulation (TMS) aims to enhance motor function and reduce spasticity in individuals with SCI by modulating cortical excitability and promoting neural plasticity (Tazoe & Perez, 2015). Despite promising results in clinical studies, limitations such as small sample sizes and variability in patient responses underscore the need for larger, controlled trials to establish its efficacy and optimize treatment protocols (Tazoe & Perez, 2015). Nevertheless, TMS holds potential as an adjunct therapy to rehabilitation for improving motor recovery and functional outcomes in SCI patients (Tazoe & Perez, 2015).
Complementary Therapies
Artificial Intelligence
Another new route of research being explored in regards to SCI repair is the use of Artificial Intelligence (AI). Through the creation of personalized treatment plans and real-time monitoring, AI has helped revolutionize rehabilitation strategies. AI algorithms currently work to analyze patient-specific data, such as electromyography (EMG) signals and motor function assessments, helping with the optimization of rehabilitation protocols in a dynamic manner (Murphy & Thomas, 2023).
A clinical study conducted by Murphy & Thomas (2023), a cohort of 50 SCI patients had AI-guided rehabilitation programs implemented for them. The AI system used machine learning algorithms to continuously monitor and adjust exercise intensity and frequency based on real-time EMG feedback. The personalization of this approach resulted in a 30% increase in motor function recovery as compared to the conventional rehabilitation methods currently in place through the BBB locomotor rating scale (Murphy & Thomas, 2023).
Moreover, AI-driven predictive models accurately forecasted motor recovery trajectories in 85% of SCI patients, allowing for the use of early intervention strategies and personalized treatment plans (Yagi et al., 2023). These models used demographic data (age, gender, etc.), injury severity metric (level and completeness of injury), and neuroimaging findings (such as MRI scans) to predict individualized outcomes. For example, patients with less severe injuries and younger age profiles often responded more favorably to tailored rehabilitation protocols, emphasizing how important AI is for optimizing rehabilitation outcomes for SCI patients.
Optogenetics
Optogenetics is a biological technique that involves the use of light to control cells within living tissue, typically neurons, that have been genetically modified to express light-sensitive ion channels. This method allows researchers to precisely manipulate the activity of specific neurons in order to study their functions and roles in various neural circuits and behaviors. As they offer precise control over neural circuits within the spinal cord, presenting novel therapeutic avenues for restoring sensory and motor functions in SCI patients, they allow researchers to selectively activate or inhibit specific neural pathways involved in motor control and sensory perception (Deng et al., 2021).
In preclinical trials conducted by Deng et al. (2021), optogenetic activation of corticospinal neurons in rodent models of SCI led to a 50% improvement in motor function scores as measured by the BBB locomotor rating scale (Deng et al., 2021). Optogenetic stimulation involves the delivery of light pulses to activate opsins expressed in corticospinal neurons, thereby enhancing their neuronal excitability and promoting motor recovery. On the other hand, optogenetic inhibition of inhibitory interneurons in SCI animals resulted in reduced spasticity and improved motor coordination, highlighting the potential of optogenetics in modulating neural circuits to improve the functional outcomes in SCI.
Discussion - Spinal Cord Injury Repair
This review discussed the various aspects of spinal cord injury repair research, specifically focusing on the regenerative, neuroprotective and complementary therapies. Researchers aimed to exemplify the feasibility of novel approaches, considering the cascading events that occur during pathophysiology of SCI, multicellular and multimolecular interactions and promising treatments for the neuroprotection, immunomodulation and neuro-regeneration of spinal cord. Several recent approaches were more successful than others in improving motor function outcomes (Figure 3). This graph has only included the therapies that are backed by statistical data, hence more theoretical methods have not been included. For pharmacological interventions, the data from NACA and growth factors is averaged, and for stem cell therapies, the data from MSCs and NPCSs is averaged. The graph compares the percentage improvement in motor function across different therapies, including MSC transplantation, NPC transplantation, exosome therapy, TMS, biomaterial scaffolds, pharmacological interventions, AI rehabilitation, and optogenetics.
Optogenetics demonstrated the highest improvement in motor function with a 50% enhancement in motor function recovery, followed by pharmacological intervention by 46.5% while TMS is the lowest in improving motor function by 20%. It should be noted that there has been limited research done in the field of optogenetics, and this paper only takes into account a few papers regarding this topic, presenting a potential bias. Pharmacological intervention and optogenetics are much more effective due to their targeted approaches to mitigate specific cellular damage, control specific neural circuits, and help support tissue regeneration. NACA reduces the oxidative stress and inflammation and optogenetics provides precise neural control. Furthermore, preclinical trials by Deng et al. (2021) demonstrated that optogenetic activation of corticospinal neurons in rodent models led to a 50% improvement in motor function scores. In contrast, TMS has far less of a direct impact on the spinal cord and is typically used as adjunctive therapy, so it is not wholly successful independently.
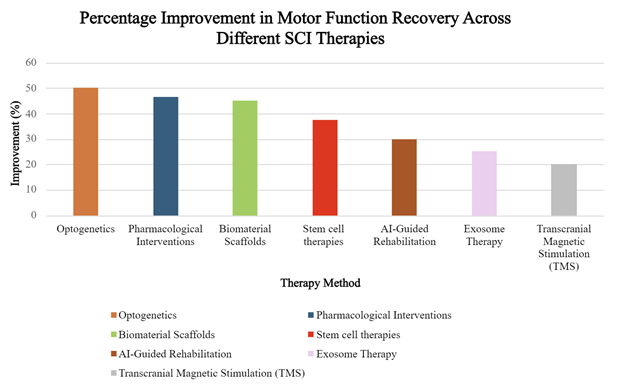
Figure 3. Overview of Improvement in Motor Function Recovery Across Different SCI Therapies Figure based on summary of key findings from current review. (Deng et al., 2021; Fang et al., 2017; Isaković et al., 2023; Murphy & Thomas, 2023; Pandya et al., 2014; Tazoe & Perez, 2015; Wang et al., 2018; Wilcox et al., 2014; Zhang et al., 2023).
Stem cell therapy is a focal point of SCI research due to its regenerative potential and ability to modulate immune responses. MSCs, derived from various sources including human umbilical cords, have shown some positive results in preclinical models and early-phase clinical trials (Isaković et al., 2023; Fan et al., 2022). Other innovative approaches such as exosome therapy have shown good results in preclinical studies, showing a decrease in inflammatory markers and an increase in motor function. While AI allows for further personalization and prediction of treatments and outcomes of SCI repair, but more research is required to determine how best to utilize it for SCI repair (Murphy & Thomas, 2023).
Despite multiple scientific research advancements for SCI, there were still several challenges that remained in the treatment in the SCI. Immune rejection, limited cell survival, and the complexity of the injury environment were big hurdles to the clinical translation of stem cell therapies. Also, variability in patient responses and the long-term safety of these treatments need to be thoroughly investigated. Regarding the popular approach of stem cell therapy, the stem cells that are used often need to be taken from a human embryo and raises questions regarding ethical and social concerns. One major issue regards the time in which an embryo can be considered a human. Rules regarding the harvest and use of stem cells can only be set once all parties come to a consensus on this issue. Exosome therapy is also quite new, and the scalability and standardization of exosome production also poses significant challenges that must be addressed before widespread clinical application can be realized (Schepici et al., 2023). Furthermore, pharmacological interventions need to be further investigated to determine optimal dosage regimens and long-term safety profiles, as the usage of drugs and their effects must be carefully evaluated (Schepici et al., 2023). AI also introduces concerns about data privacy, algorithmic bias, and the potential for over-reliance on automated systems, so therefore careful frameworks, transparency, and continuous monitoring are needed to prevent disparities in treatment outcomes (Murphy & Thomas, 2023). Finally, optogenetics have ethical concerns related to genetic modification and the long-term effects of neuronal manipulation (Deng et al., 2021). In brief, developing and testing new therapies is crucial for the progression of SCI repair.
While research is progressing rapidly, many treatments are unable to be applied clinically. Clinical trials regarding SCI have increasing low enrollment facing challenges including but not limited to low incidence of injury, variable severity, highly debatable approaches to therapeutic intervention and high cost of enrolled participants (Mulcahey et al., 2020). Even beyond difficulties related to completing clinical trials, there are also major gaps in clinical trial reporting. In 2021, a study was conducted on a subset of data extracted from SCI clinical trials. It showed that only 11.2% of trials correctly identified their study type, provided valid study status, and provided sufficient detail about injury characteristics (Warner et al., 2021). Another noteworthy figure is that although almost half of the review clinical trials were marked as completed, 75.4% had no results available to the public (Warner et al., 2021).
Furthermore, limitation of the rodent models for predicting efficacy in humans because of the varying anatomy and size of the different models also impeded translational efficiency (Tian et al., 2023). This means that differing animal models that better represent human spinal cords, such as primates, should be used to better predict the efficacy and limitations in the human model (Tian et al., 2023). Also, for better success in clinical trials, the preclinical trials should attain more extensive data to minimize adverse effects in the clinical stage, replicate the preclinical findings in a third-party laboratory to ensure its efficacy, to use large-scale randomized trials to better observe the efficacy and long-term effects of the treatment, and use multi-targeted, combinatorial approaches (Sahni & Kessler, 2010). Yet, there is a continued hope that spinal cord injury research will continue to develop further and create treatments that will be beneficial to those suffering from SCIs.
Conclusion and Future Directions
The advancements in SCI research highlighted in this review underscore the potential of various therapeutic approaches in improving motor function recovery. Pharmacological interventions have shown the most promising results and optogenetics, with significant enhancements in motor and sensory-motor functions. This review provides an overall comprehensive look into the most mainstream treatments for SCI including current progress, limitations, and research that has been completed regarding these treatments. SCI repair research is an ongoing process that still requires extensive research and effort.
Future research regarding SCI should focus largely on increasing the amount of research, improving the transparency of data and standardizing data collection. The biggest challenge facing SCI repair is the lack of standardized research. It is crucial that the scientific community can see the results of clinical trials. Working with incomplete datasets can directly contribute to the redundancy of clinical trials and further slow down the process of developing a cure. Scientists will need to find a way to properly share the research they have done, whether that be through a new dedicated platform or creating a new organization dedicated to the cause. Knowledge regarding SCI is seemingly filled with caveats and contradictions that makes the overall SCI research scene incredibly difficult to navigate. Although the process of conducting and testing repair methods for SCI may never be fully standardized due to the erratic nature of SCI, scientists should still work towards setting more definitive baselines regarding research for SCI.
To conclude, SCI treatment is at a pivotal stage, with many therapies currently being under investigation. Research and collaboration is important for further advancements that can be translated into clinical treatments for SCI patients. Collectively, these advancements will pave the way for more effective and personalized treatments for SCI, bringing hope for improved quality of life for affected individuals.
References
Aderinto, N., Abdulbasit, M. O., & Olatunji, D. (2023). Stem cell-based combinatorial therapies for spinal cord injury: a narrative review of current research and future directions. Annals of Medicine and Surgery (2012), 85(8), 3943–3954. https://doi.org/10.1097/MS9.0000000000001034
Ahuja, C. S., Wilson, J. R., Nori, S., Kotter, M. R. N., Druschel, C., Curt, A., & Fehlings, M. G. (2017). Traumatic spinal cord injury. Nature Reviews Disease Primers, 3(1), 17018.
Bennett, J., Das, J. M., & Emmady, P. D. (2024, March 10). Spinal cord injuries. In StatPearls [Internet]. StatPearls Publishing. https://www.ncbi.nlm.nih.gov/books/NBK560721/
Chen, Y., Tang, Y., Vogel, L. C., & Devivo, M. J. (2013). Causes of spinal cord injury. Topics in Spinal Cord Injury Rehabilitation, 19(1), 1–8. https://doi.org/10.1310/sci1901-1
Costăchescu, B., Niculescu, A. G., Dabija, M. G., Teleanu, R. I., Grumezescu, A. M., & Eva, L. (2022). Novel strategies for spinal cord regeneration. International Journal of Molecular Sciences, 23(9), 4552. https://doi.org/10.3390/ijms23094552
Deng, W., Wu, G., Min, L., Feng, Z., Chen, H., Tan, M., Sui, J., Liu, H., & Hou, J. (2021). Optogenetic neuronal stimulation promotes functional recovery after spinal cord injury. Frontiers in Neuroscience, 15, 640255.
Dietz, V. A., Roberts, N., Knox, K., Moore, S., Pitonak, M., Barr, C., Centeno, J., Leininger, S., New, K. C., Nowell, P., Rodreick, M., Geoffroy, C. G., Stampas, A., & Dulin, J. N. (2022). Fighting for recovery on multiple fronts: The past, present, and future of clinical trials for spinal cord injury. Frontiers in Cellular Neuroscience, 16, 977679.
Fan, B., Wei, Z., & Feng, S. (2022). Progression in translational research on spinal cord injury based on microenvironment imbalance. Bone Research, 10(1). https://doi.org/10.1038/s41413-022-00199-9
Fang, H., Liu, C., Yang, M., Li, H., Zhang, F., Zhang, W., & Zhang, J. (2017). Neurotrophic factor and Trk signaling mechanisms underlying the promotion of motor recovery after acute spinal cord injury in rats. Experimental and Therapeutic Medicine, 14, 652-656. https://doi.org/10.3892/etm.2017.4516
U.S. Food and Drug Administration. (n.d.). Step 3: Clinical Research. FDA. Retrieved from https://www.fda.gov/patients/drug-development-process/step-3-clinical-research
Guo, W., Zhang, X., Zhai, J., & Xue, J. (2022). The roles and applications of neural stem cells in spinal cord injury repair. Frontiers in Bioengineering and Biotechnology, 10, 966866. https://doi.org/10.3389/fbioe.2022.966866
Isaković, J., Šerer, K., Barišić, B., & Dinko Mitrečić. (2023). Mesenchymal stem cell therapy for neurological disorders: The light or the dark side of the force? Frontiers in Bioengineering and Biotechnology, 11, 1139359. doi: 10.3389/fbioe.2023.1139359
Liu, Y., Yang, X., He, Z., Li, J., Li, Y., Wu, Y., Manyande, A., Feng, M., & Xiang, H. (2023). Spinal cord injury: global burden from 1990 to 2019 and projections up to 2030 using Bayesian age-period-cohort analysis. Frontiers in Neurology, 14, 1304153. doi: 10.3389/fneur.2023.1304153
Mazzini, L., Gelati, M., Profico, D. C., Sorarù, G., Ferrari, D., Copetti, M., Muzi, G., Ricciolini, C., Carletti, S., Giorgi, C., Spera, C., Frondizi, D., Masiero, S., Stecco, A., Cisari, C., Bersano, E., Marchi, F. D., Sarnelli, M. F., Querin, G., & Cantello, R. (2019). Results from Phase I clinical trial with intraspinal injection of neural stem cells in amyotrophic lateral sclerosis: A long‐term outcome. STEM CELLS Translational Medicine, 8(9), 887–897. https://doi.org/10.1002/sctm.18-0154
Mulcahey, M. J., Jones, L. A. T., Rockhold, F., Rupp, R., Kramer, J. L. K., Kirshblum, S., Blight, A., Lammertse, D., Guest, J. D., & Steeves, J. D. (2020). Adaptive trial designs for spinal cord injury clinical trials directed to the central nervous system. Spinal Cord, 58(12), 1235–1248. https://doi.org/10.1038/s41393-020-00547-8
Murphy, C., & Thomas, F. P. (2023). Generative AI in spinal cord injury research and care: Opportunities and challenges ahead. Journal of Spinal Cord Medicine, 46(3), 341–342.[WSA3]
Pandya, J. D., Readnower, R. D., Patel, S. N., Yonutas, H. M., Pauly, J. R., Goldstein, G. D., Rabchevsky, A. G., & Sullivan, P. F. (2014). N-acetylcysteine amide confers neuroprotection, improves bioenergetics and behavioral outcome following TBI. Experimental Neurology, 257, 106–113.
Roberts, T. T., Leonard, G. R., & Cepela, D. J. (2016). Classifications in brief: American spinal injury association (ASIA) impairment Scale. Clinical Orthopaedics and Related Research, 475(5), 1499–1504.
Sahni, V., & Kessler, J. A. (2010). Stem cell therapies for spinal cord injury. Nature Reviews: Neurology, 6(7), 363–372.
Scheff, S. W., Saucier, D. A., & Cain, M. E. (2002). A statistical method for analyzing rating scale data: The BBB Locomotor Score. Journal of Neurotrauma, 19(10), 1251–1260.
Schepici, G., Silvestro, S., & Mazzon, E. (2023). Regenerative effects of exosomes-derived MSCs: An overview on spinal cord injury experimental studies. Biomedicines, 11(1), 201.
Shao, A., Tu, S., Lu, J., & Zhang, J. (2019). Crosstalk between stem cell and spinal cord injury: pathophysiology and treatment strategies. Stem Cell Research & Therapy, 10(1), 238. https://doi.org/10.1186/s13287-019-1357-z
Son, D., Zheng, J., Kim, I. Y., Kang, P. J., Park, K., Priscilla, L., Hong, W., Yoon, B. S., Park, G., Yoo, J. E., Song, G., Lee, J. B., & You, S. (2023). Human induced neural stem cells support functional recovery in spinal cord injury models. Experimental & Molecular Medicine, 55(6), 1182–1192. https://doi.org/10.1038/s12276-023-01003-2
Spinal cord injury. (n.d.). National Institute of Neurological Disorders and Stroke. https://www.ninds.nih.gov/health-information/disorders/spinal-cord-injury
Spinal cord injury - Symptoms and causes - Mayo Clinic. (2023, October 12). Mayo Clinic. https://www.mayoclinic.org/diseases-conditions/spinal-cord-injury/symptoms-causes/syc-20377890
Tazoe, T., & Perez, M. A. (2015). Effects of repetitive transcranial magnetic stimulation on recovery of function after spinal cord injury. Archives of Physical Medicine and Rehabilitation, 96(4), S145–S155.
Thuret, S., Moon, L. D., & Gage, F. H. (2006). Therapeutic interventions after spinal cord injury. Nature Reviews: Neuroscience, 7(8), 628–643. https://doi.org/10.1038/nrn1955
Tian, T., Zhang, S., & Yang, M. (2023). Recent progress and challenges in the treatment of spinal cord injury. Protein & Cell, 14(9), 635-652.
Traumatic Spinal Cord Injury Facts and Figures at a Glance 2024 SCI Data Sheet. (2024). National Spinal Cord Injury Statistical Center. https://www.nscisc.uab.edu/public/Facts%20and%20Figures%202024%20-%20Final.pdf
Wang, L., Gu, S., Gan, J., Tian, Y., Zhang, F., Zhao, H., & Lei, D. (2021). Neural stem cells overexpressing nerve growth factor improve functional recovery in rats following spinal cord injury via modulating microenvironment and enhancing endogenous neurogenesis. Frontiers in Cellular Neuroscience, 15, 773375. https://doi.org/10.3389/fncel.2021.773375
Wang, Y., Tan, H., & Hui, X. (2018). Biomaterial scaffolds in regenerative therapy of the central nervous system. BioMed Research International, 2018, 1–19.
Warner, F.M., Bailey, N.G., Scheuren, P.S. et al. (2021). Clinical trials and tribulations: lessons from spinal cord injury studies. Spinal Cord 59, 1256–1260. https://doi.org/10.1038/s41393-021-00699-1
Wilcox, J. T., Satkunendrarajah, K., Zuccato, J. A., Nassiri, F., & Fehlings, M. G. (2014). Neural precursor cell transplantation enhances functional recovery and reduces astrogliosis in bilateral compressive/contusive cervical spinal cord injury. STEM CELLS Translational Medicine, 3(10), 1148–1159. https://doi.org/10.5966/sctm.2014-0029
World Health Organization. (2024, April 16). Spinal cord injury. Who.int; World Health Organization: WHO. https://www.who.int/news-room/fact-sheets/detail/spinal-cord-injury
Xue, X., Shu, M., Xiao, Z., Zhao, Y., Li, X., Zhang, H., Fan, Y., Wu, X., Chen, B., Xu, B., Yang, Y., Liu, W., Liu, S., & Dai, J. (2021). Lineage tracing reveals the origin of Nestin-positive cells are heterogeneous and rarely from ependymal cells after spinal cord injury. Science China Life Sciences, 65(4), 757–769.
Yagi, M., Yamanouchi, K., Fujita, N., Funao, H., & Ebata, S. (2023). Revolutionizing spinal care: current applications and future directions of artificial intelligence and machine learning. Journal of Clinical Medicine, 12(13), 4188.
Yang, B., Zhang, F., Cheng, F., Ying, L., Wang, C., Shi, K., Wang, J., Xia, K., Gong, Z., Huang, X., Yu, C., Li, F., Liang, C., & Chen, Q. (2020). Strategies and prospects of effective neural circuits reconstruction after spinal cord injury. Cell Death & Disease, 11(6), Article 439. https://doi.org/10.1038/s41419-020-2620-z
Zhang, X., Jiang, W., Lu, Y., Mao, T., Gu, Y., Ju, D., & Dong, C. (2023). Exosomes combined with biomaterials in the treatment of spinal cord injury. Frontiers in Bioengineering and Biotechnology, 11, 1077825. https://doi.org/10.3389/fbioe.2023.1077825
Comentarios